News | January 13, 2013
Improving climate change predictions, one cloud at a time
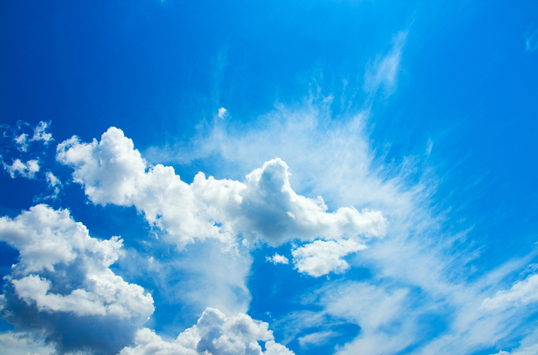
By Megan Scudellari,
Jet Propulsion Laboratory
Clouds are currently the greatest source of uncertainty in climate change predictions — but not for long. By modeling the complexity of clouds at small scales, researchers at NASA’s Jet Propulsion Laboratory are working to improve climate prediction models in a big way.
By the end of this century, the Earth’s surface will warm between 2 and 4 degrees Celsius (roughly 3 to 7 degrees Fahrenheit), according to the Intergovernmental Panel on Climate Change (IPCC). That may not sound like much, but even a single degree fluctuation in Earth’s temperature could have unpredictable consequences on weather patterns, sea levels, animal habitats and more. So atmospheric scientists are working around the clock to narrow the range reported by the IPCC in the hopes of better forecasting the future. And the best way to do that, it turns out, is in the clouds."By understanding more about the physics of individual clouds, we can help refine weather and climate models."
Clouds are the single largest source of uncertainty in predictions of climate change, the IPCC declared in 2007. While some climate models — mathematical representations of the physical forces that shape climate — depict an increase in cloud cover by the year 2100, others show a decrease because of the difficulty of accurately representing clouds. “We just don’t know what will happen to the clouds,” says Joao Teixeira, Deputy Director of the NASA Jet Propulsion Laboratory’s Center for Climate Sciences.
Modeling Small Scale Turbulence from Center for Climate Sciences, JPL on Vimeo.
Today, Teixeira and colleagues at the Jet Propulsion Laboratory (JPL) are on the hunt to find out. Recently, JPL researchers demonstrated the ability to improve large climate change model predictions by simulating the formation of clouds on small scales, at higher resolution than ever before. This effort, to emphasize the trees in order to better see the forest, could help us better understand the future of the planet on which we live.
“Smaller scale models are very much a path toward improving our large scale models,” says Chris Bretherton, a professor of atmospheric science and applied mathematics at the University of Washington, who was not involved in the research. “In 20 years, we may be getting close to simulating individual clouds in a global model.”
Clouds in a box
Clouds cover about 70 percent of the Earth's surface and have an enormous effect on climate: Some clouds, especially low-lying hazy or puffy clouds, reflect sunshine and cool the planet; others, such as high, wispy clouds, trap heat emanating from Earth’s surface and warm the planet. Clouds currently have an overall cooling effect on the planet, but as the Earth heats due to global warming, the type and position of clouds could change.
Georgios Matheou is a research scientist with the Climate Physics Group at JPL.
So to predict climate change over the next 10 to 100 years, scientists need to be able to accurately predict what will happen to clouds. But despite simple ingredients — tiny droplets of water or ice crystals — clouds are extraordinarily complex entities governed by competing physical forces, and it takes a significant amount of computing power to calculate how they form and change.
“Computers are getting better and better, but still their power is limited,” says Teixeira. In fact, though scientists know the equations needed to model the physical processes that determine the formation and evolution of clouds, today’s computers can’t handle the complex calculations that are required to simulate each and every cloud that forms across the globe. Instead, atmospheric scientists resort to dividing the planet into large boxes, or “grid cells,” ranging from 100 to 300 kilometers (60 to 190 miles) on each side. In each of these boxes, a computer algorithm calculates a coarse estimate of cloud formation and movement. This way the calculation becomes computationally feasible, but at the expense of fine detail.
Since clouds are formed by small-scale atmospheric motions, Bretherton says, the interaction and combination of the different atmospheric motions – small and large – is difficult to be accurately represented in the large grid boxes. Because of this, clouds are not realistically simulated in current weather and climate models.
But Georgios Matheou, a research scientist with the Climate Physics Group at JPL, has recently made improvements in how clouds are represented in climate models by starting small and thinking big. “By understanding more about the physics of individual clouds, we can help refine weather and climate models,” says Matheou.
Modeling turbulence
Together with Daniel Chung, a former JPL postdoctoral fellow and now a lecturer in mechanical engineering at the University of Melbourne in Australia, Matheou divided climate model grid cells of 100-kilometers by 100-kilometers into 10-meter by 10-meter cloud boxes and again into 1-millimeter by 1-millimeter turbulence boxes, like opening a Russian doll to pull out smaller and smaller dolls from inside. In their tiny boxes, Matheou and Chung analyzed turbulence, the chaotic motion that occurs as warm air from Earth’s surface rises and mixes with the air above, forming clouds and storms.
The duo developed computer simulations of turbulence on these small scales under various degrees of stratification — in which lighter air sits atop heavier air — and shear — when the upper layer moves faster than the lower one. While shear destabilizes layers of air, stratification stabilizes them, so “it’s like a competition between the two,” says Matheou. “And we’ve tried to figure out the dynamics of this competition.”
Using one of the world’s fastest supercomputers, Pleiades at the NASA Ames Research Center in Silicon Valley, California, Matheou and Chung recorded how different levels of shear and stratification affect the amount of turbulence in a small space. They presented their results at the 64th Annual Meeting of the American Physical Society’s Division of Fluid Dynamics in Baltimore, Maryland, in November 2011, and subsequently published them in April 2012 in the Journal of Fluid Mechanics. The images produced (Figure 3) were awarded the Division of Fluid Dynamic’s 2011 Milton Van Dyke Award for artistic value, scientific content and originality.
The turbulence simulations are now being used to develop more accurate statistical representations of clouds under different atmospheric conditions in larger grid boxes. “Now we have the tools to predict the amount of turbulence without having to do the simulation,” says Matheou. Their findings will improve large-scale climate models, he says.
Scientists will continue to advance our ability to represent clouds and more accurately predict global climate change, says Teixeira. “It’s not an easy problem,” he adds, pausing. “But I’m optimistic. There are a lot of great people working on this, and good things will happen.”